Biomaterials Science
The utilization of biomaterials to increase functionality of organ-on-chip systems and improve the physiological function of diseased or damaged tissues by replacement or regeneration is imperative in life science applications. For this purpose, polymeric materials and hydrogels are formulated. Hydrogels, which can absorb large amounts of water and biological fluids, mimic natural living tissues more than many other biomaterials due to their high water content, porosity, soft structure and stability (Filiz et al., 2022; Cakmak et al. 2022). Apart from these, decellularized extracellular matrix (dECM)-based biomaterials derived from allogeneic or xenogenic tissues have recently become an eye catching research topic in tissue engineering applications due to their ability to more closely mimic natural microenvironment (Yaldiz et al. 2022). The preparation of dECM-based biomaterials consists of two main steps, including the decellularization of a tissue or organ and terminal sterilization of the dECM, respectively, and both steps are highly effective in obtaining a biomaterial with the desired properties. dECM-based biomaterials encompass mixtures of various biomolecules that regulate cell adhesion, proliferation, migration, and differentiation, such as glycosaminoglycans, adhesion proteins such as laminin, integrin and structural proteins (Yaldiz et al., 2021). Therefore, the selected decellularization and sterilization methods should have a minimal negative impact on the biochemical and morphological composition as well as mechanical properties of the decellularized matrix. Supercritical carbon dioxide application steps forward by ensuring further removal of nuclear compounds from the tissue and sterilization at the same time.


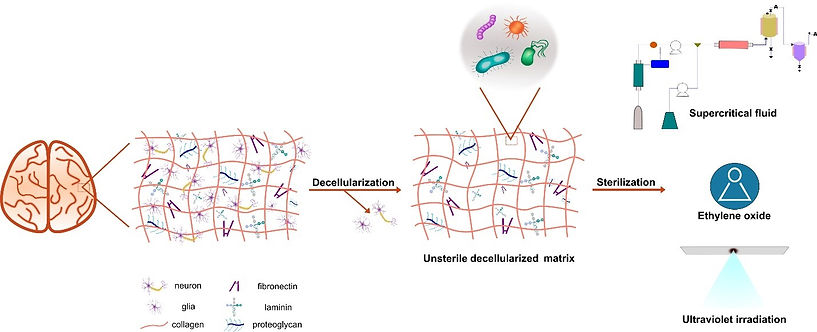
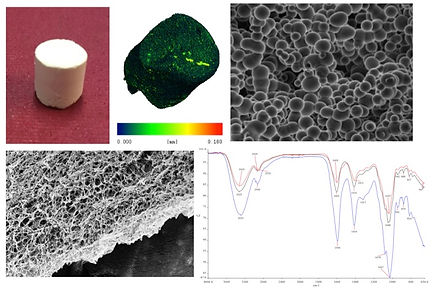
We have also tested the reusability by subjecting the treated enzyme to consecutive reactions, retreating with supercritical CO2, where four successful loops and seventeen reactions were realized (Senyay-Oncel & Yesil-Celiktas, 2013). The mechanisms behind have not been fully elucidated but intramolecular interactions, hydration of charged groups and stabilization of hydrogen bonds may play roles. A prior observation was related to the length of existing hydrogen bonds within enzymes. We have observed chemical shifts at the lowest activity sample, which were assigned to protons of S-H, O-H and N-H groups and shorter as detected by NMR. We have carried out numerous characterization tests to explain these changes and our results have made significant impact on understanding the mechanisms behind activity enhancement by high pressure.
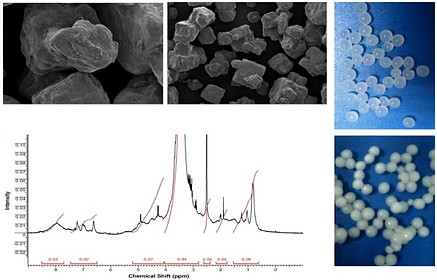
Supercritical Fluid Treated Biomaterials
High pressure applications to bioscience were demonstrated in the early 1900s by the discovery of microorganisms in deep sea sediments in the 1880s. However, studies investigating the effects of high pressure on protein type structures, microorganisms and cells were suggesting adverse effects of high pressure treatments. However, we intend to explore supercritical fluids and high pressure treatments as elicitors or activators to enhance the capability of microorganism, cells and protein type structures. The results of our project focusing on this aspect proved that under optimized conditions, supercritical CO2 can serve as a potential media for improving the activity of hydrolytic enzymes between 48.3 - 67.7 % (Senyay-Oncel & Yesil-Celiktas, 2015; Senyay-Oncel et al., 2014; Senyay-Oncel & Yesil-Celiktas, 2011).
Another completed project was dealing with alterations in material characteristics of microfluidic chips subsequent to sterilization with various techniques including supercritical fluids as a new approach (Yavuz et al., 2016).
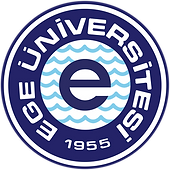

Ege University
Department of Bioengineering
35040 Bornova-Izmir/TURKEY